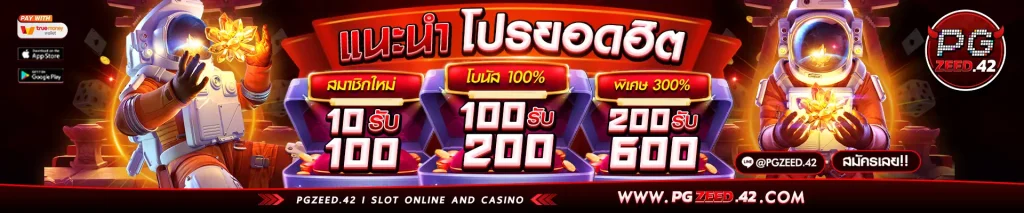
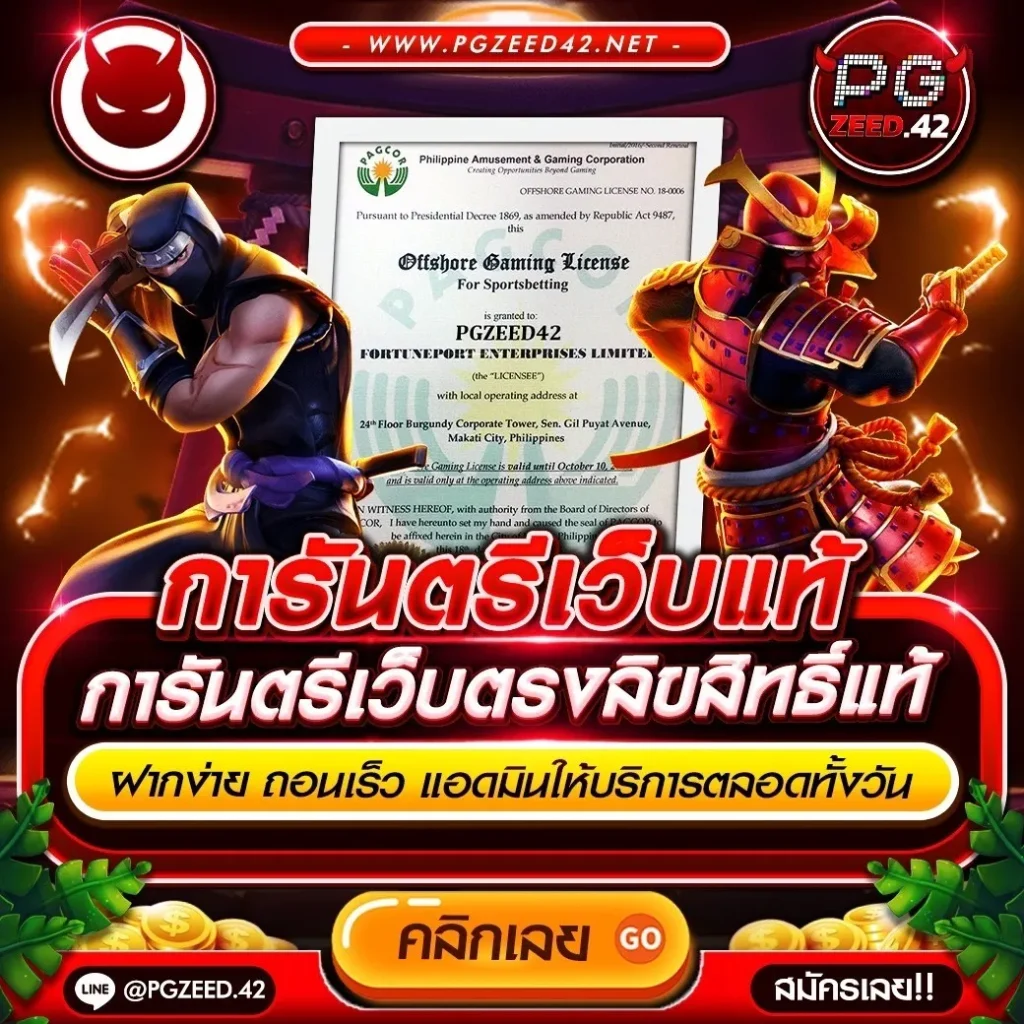
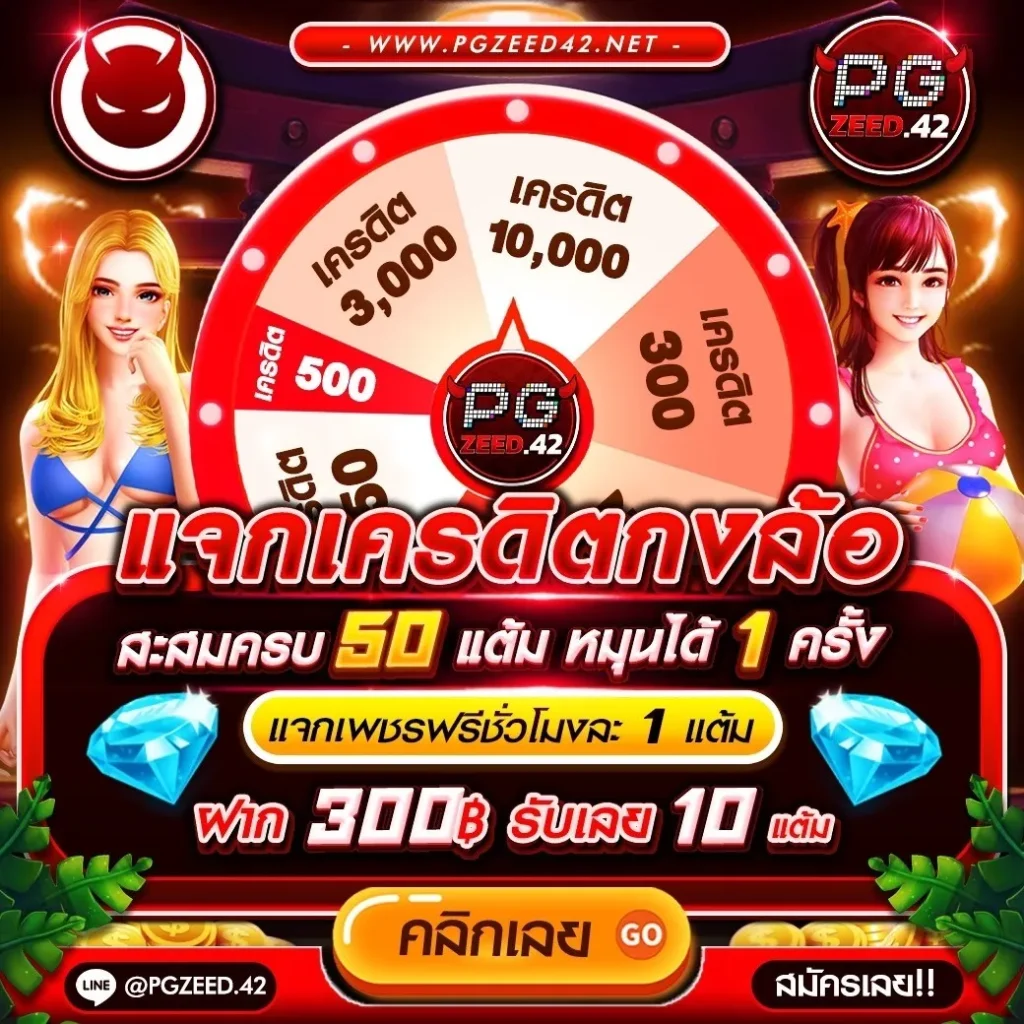
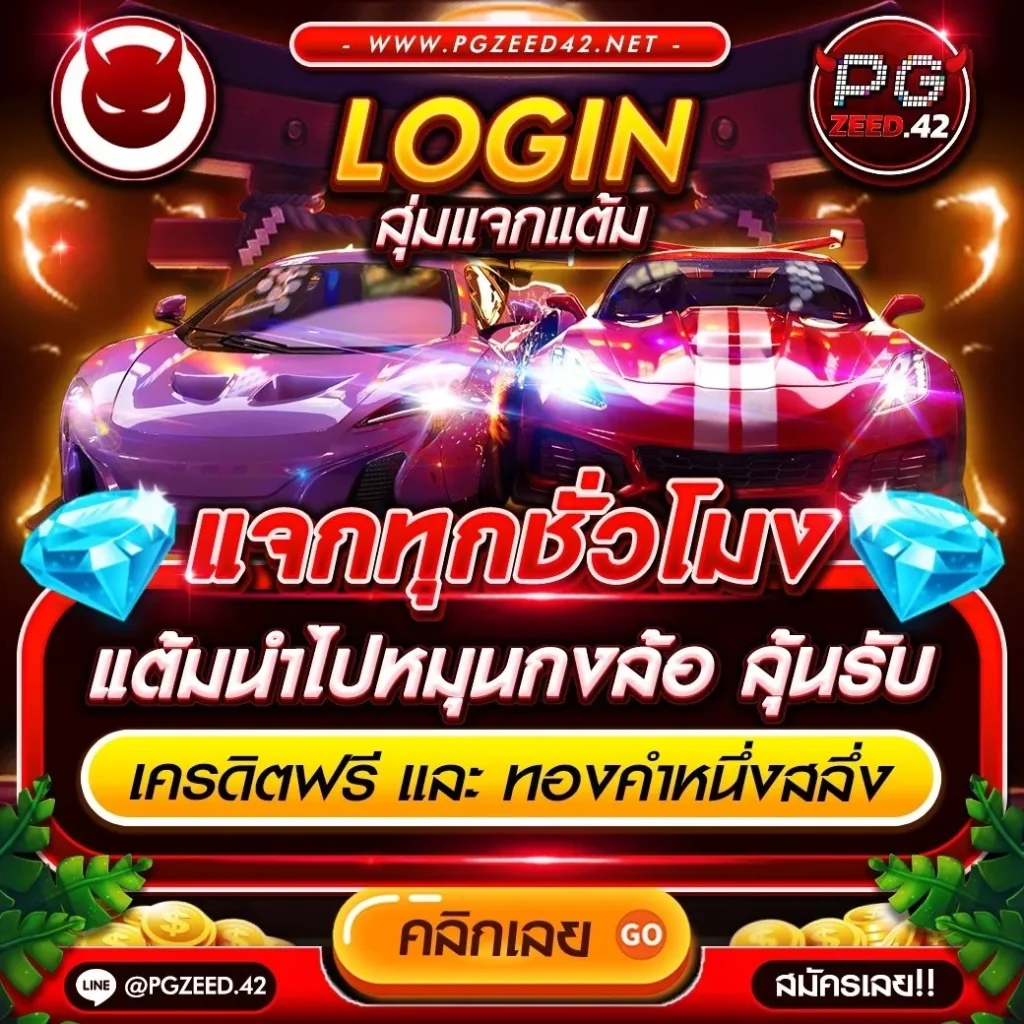
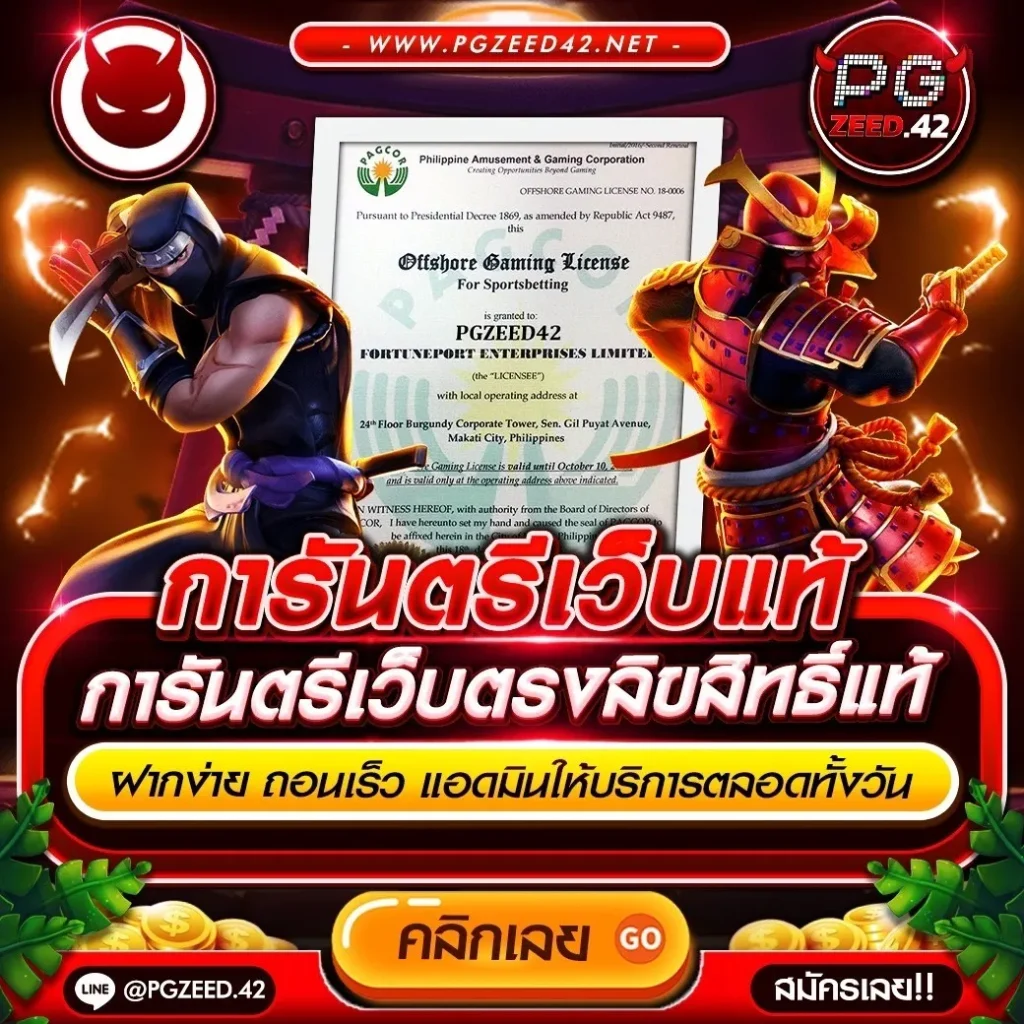
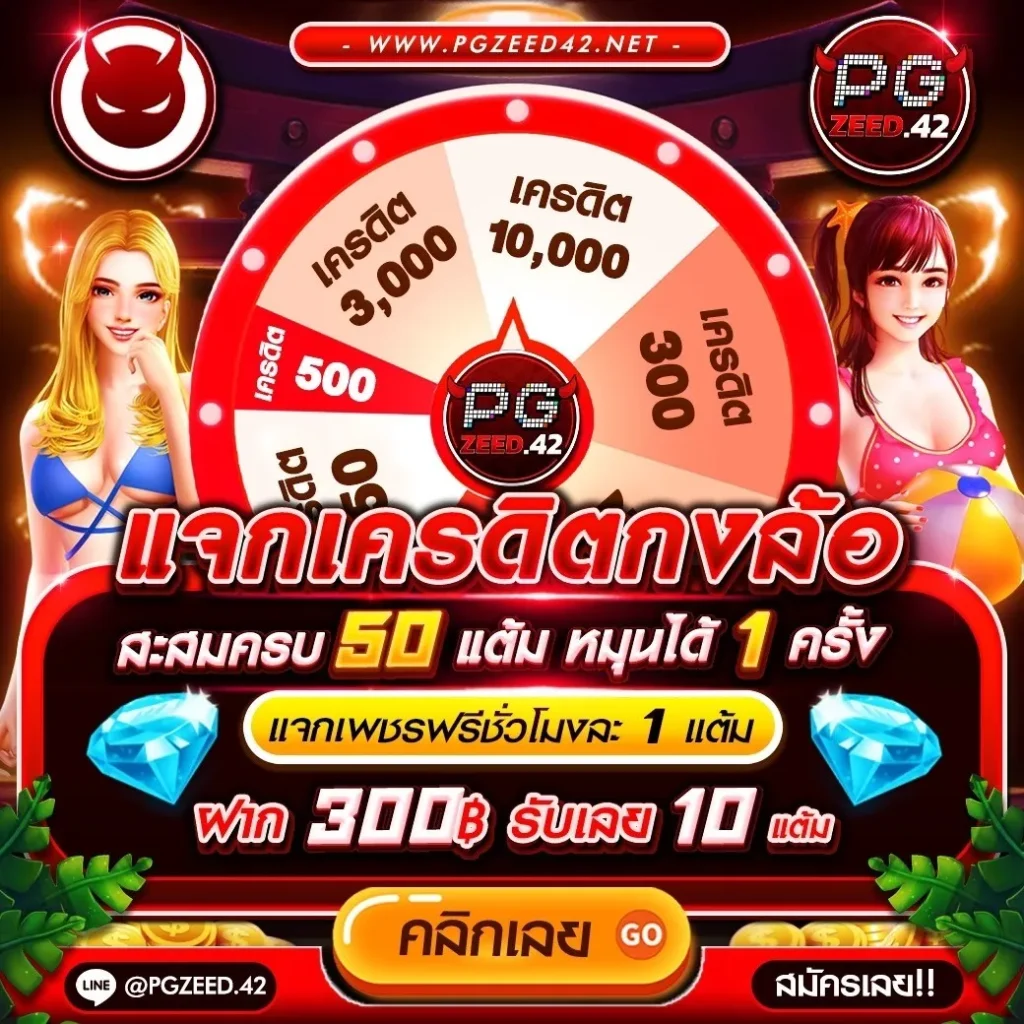
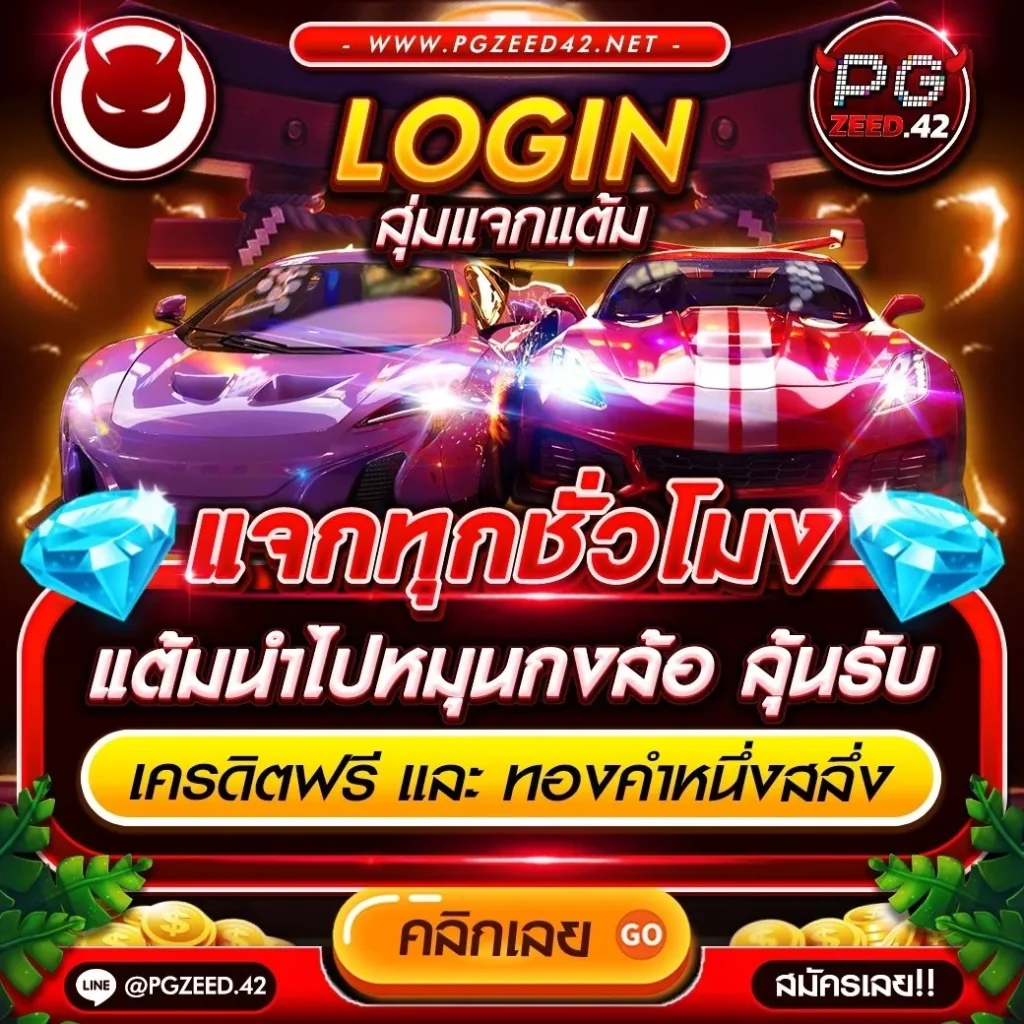
pgzeed42 เว็บตรง pg slot auto เกมสล็อตออนไลน์
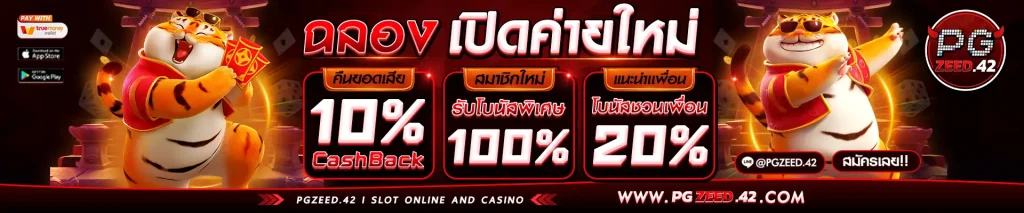
pgzeed42 ในบริบทของ PG SLOT คือแพลตฟอร์มเกมออนไลน์หรือสล็อตออนไลน์ที่เน้นการใช้นวัตกรรมและเทคโนโลยีล่าสุดในการพัฒนาเกมของตัวเอง มีจุดที่น่าสนใจมากมายเกี่ยวกับการใช้นวัตกรรมใหม่ในเกมรถสล็อต PG เช่น กราฟิกที่สมจริง ระบบเสียงที่ออกแบบมาเพื่อยกระดับประสบการณ์การเล่นเกม หรือการใช้เทคโนโลยี เช่น AI และ VR เพื่อนำมิติใหม่มาสู่เกมให้ยืม ซึ่งรวมถึงการใช้เทคโนโลยีบล็อกเชนหรือระบบการชำระเงินดิจิทัลอื่นๆ
เล่นสล็อตที่ pgzeed42 com แพลตฟอร์มสล็อตออนไลน์ มีทั้งโชคและกลยุทธ์ในการเล่น แม้ว่าจะไม่มีเคล็ดลับใดรับประกันการชนะได้ 100% เนื่องจากสล็อตส่วนใหญ่ใช้ RNG (Random Number Generator) ซึ่งจะสุ่มผลลัพธ์ของการหมุนแต่ละครั้ง แต่ก็มีวิธีที่คุณสามารถใช้เพื่อเพิ่มโอกาสในการชนะได้ ทำความเข้าใจกับไลน์ที่ชนะ สัญลักษณ์พิเศษ และรอบโบนัส ก่อนที่คุณจะเริ่มเล่น ให้ตั้งงบประมาณของคุณและยึดมั่นในงบประมาณนั้น
หลีกเลี่ยงการพนันเกินขีดจำกัดที่คุณตั้งไว้ RTP หรือ Return to Player คือเปอร์เซ็นต์ที่เกมส่งคืนให้กับผู้เล่นในระยะยาว pg slots auto เลือกเกมที่มี RTP สูงเพื่อเพิ่มโอกาสของคุณ หาก PGZEED 42 มีโบนัสหรือโปรโมชั่น: ใช้ในวันที่กำหนดเพื่อเพิ่มยอดเงินของคุณ เลือกเกมที่คุณคุ้นเคยหรือมีประสบการณ์ เพื่อให้ท่านมีความเข้าใจคุณสมบัติของเกมและรอบโบนัสเป็นอย่างดี
pgzeed 42 พร้อมโบนัสแจกมากมายในวันนี้
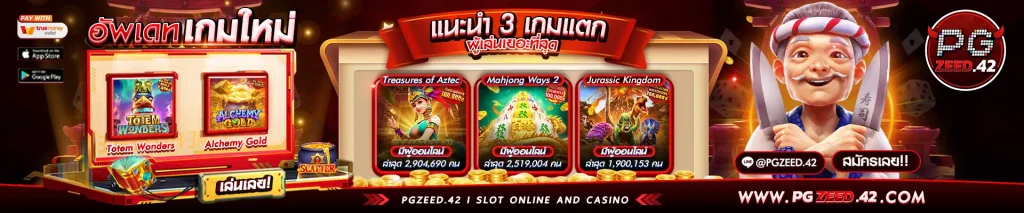
หากต้องการเล่น PG SLOT Auto ผ่านเว็บไซต์ pgzeed42 คุณต้องทำตามขั้นตอนง่ายๆ ไม่กี่ขั้นตอน ซึ่งโดยปกติจะรวมถึงการลงทะเบียน การเข้าสู่ระบบ และเริ่มเกม PG SLOT Auto คือระบบที่ให้ผู้เล่นสล็อตออนไลน์สามารถเล่นเกมได้อย่างง่ายดายและอัตโนมัติผ่านเว็บไซต์หรือแอพพลิเคชั่น ออกแบบมาเพื่อให้ผู้เล่นเข้าถึงเกมได้อย่างรวดเร็ว ไม่ว่าจะเป็นผ่านทางเว็บไซต์หรือแอปพลิเคชันบนมือถือ PGZeed มีรูปแบบการเล่นที่เรียบง่ายที่สามารถเข้าถึงได้ทั้งผู้เริ่มต้นและมืออาชีพ ด้วยการหมุนวงล้อและการรวมสัญลักษณ์ต่าง ๆ
ระบบสามารถอำนวยความสะดวกในการลงทะเบียนและฝากเงินทำให้ผู้เล่นสามารถเริ่มเล่นได้ทันทีโดยไม่มีขั้นตอนที่ซับซ้อน มีขั้นตอนการถอนเงินที่ง่ายและรวดเร็วซึ่งช่วยให้ผู้เล่นถอนเงินรางวัลออกจากเกมได้โดยไม่ต้องยุ่งยาก ระบบ Pgzeed มีมาตรฐานความปลอดภัยสูงเสมอเพื่อปกป้องข้อมูลและทรัพย์สินของผู้เล่น หากคุณมีปัญหาหรือข้อสงสัยเกี่ยวกับการใช้งานระบบสามารถติดต่อฝ่ายบริการลูกค้าได้ตลอดเวลา
จุดเด่น และ ข้อดี ของ pgzeed42 มีอะไรบ้าง
- PGZEED 42 มีคอลเลกชั่นสล็อตแมชชีนที่หลากหลาย pgzeed42 สิ่งนี้ทำให้ผู้เล่นสามารถเล่นเกมมากมายที่เหมาะกับความชอบและสไตล์ของพวกเขา ไม่ว่าจะเป็นแนวคลาสสิค ผจญภัย แฟนตาซี หรืออะไรก็ตาม
- กราฟิกและเอฟเฟกต์ที่น่าทึ่ง สล็อตบน PGZEED 42 มีการออกแบบกราฟิกที่สวยงามและเอฟเฟกต์สมัยใหม่ สิ่งนี้มอบประสบการณ์ที่ยากจะลืมเลือนให้กับผู้เล่นที่นอกเหนือไปจากเครื่องสล็อตแบบเดิมๆ
- ระบบการเล่นเกมที่เสถียรและเข้ากันได้กับโทรศัพท์มือถือ ผู้เล่นสามารถเข้าถึงและเพลิดเพลินกับเกมได้อย่างง่ายดายโดยไม่มีปัญหาการเชื่อมต่อหรือความล่าช้า
- โบนัสและโปรโมชั่น Pgzeed มักจะเสนอโบนัสและโปรโมชั่นที่น่าสนใจ เช่น ฟรีสปิน โบนัสเงินฝาก และโปรโมชั่นพิเศษอื่น ๆ สิ่งนี้จะเพิ่มโอกาสในการชนะ
- เว็บไซต์โดยตรงด้านความปลอดภัยและความน่าเชื่อถือ เช่น PGZEED24 มักจะมีมาตรฐานความปลอดภัยสูง ทั้งนี้เพื่อให้แน่ใจว่าข้อมูลส่วนบุคคลและธุรกรรมทางการเงินของผู้เล่นได้รับการคุ้มครองอย่างดี
- การบริการลูกค้าที่เป็นเลิศ มีทีมสนับสนุนลูกค้าที่ยินดีช่วยเหลือคุณในทุกปัญหาหรือข้อสงสัย อย่างรวดเร็วและมีประสิทธิภาพ
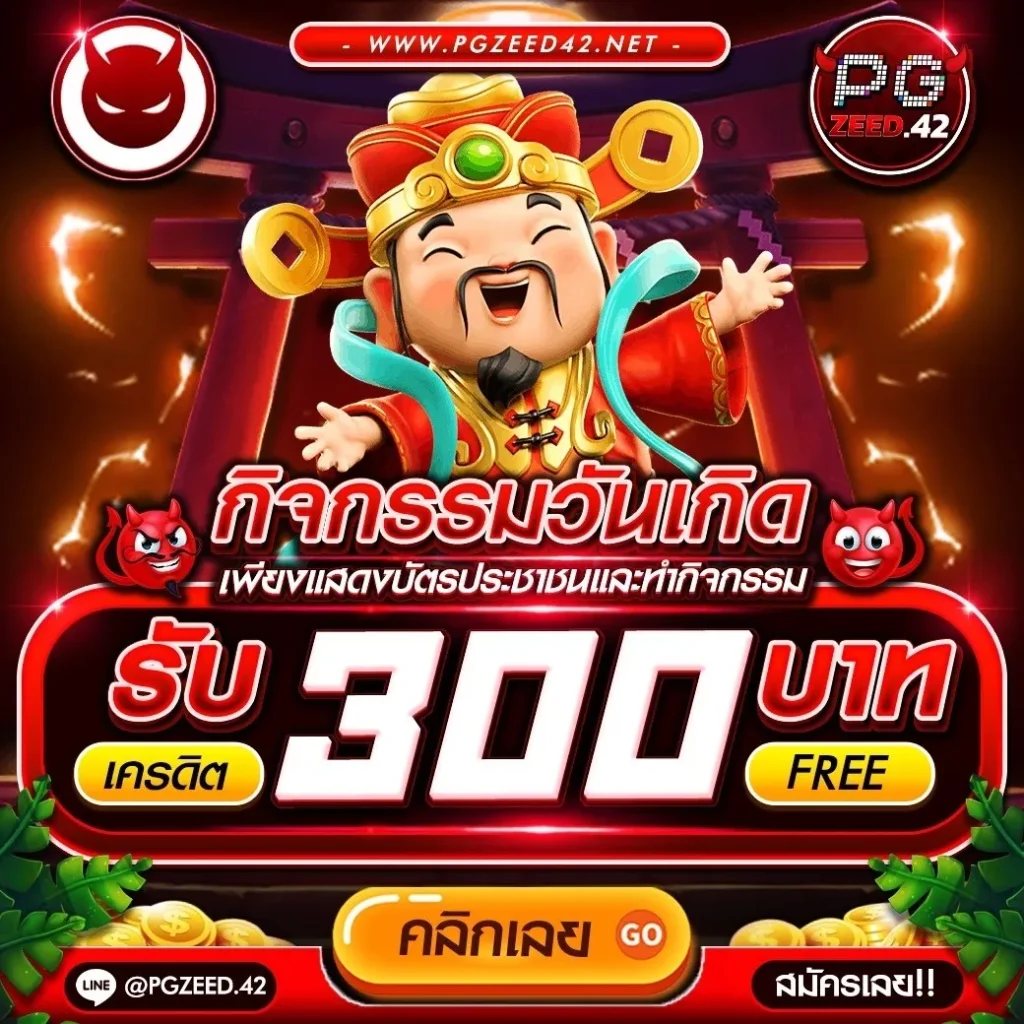
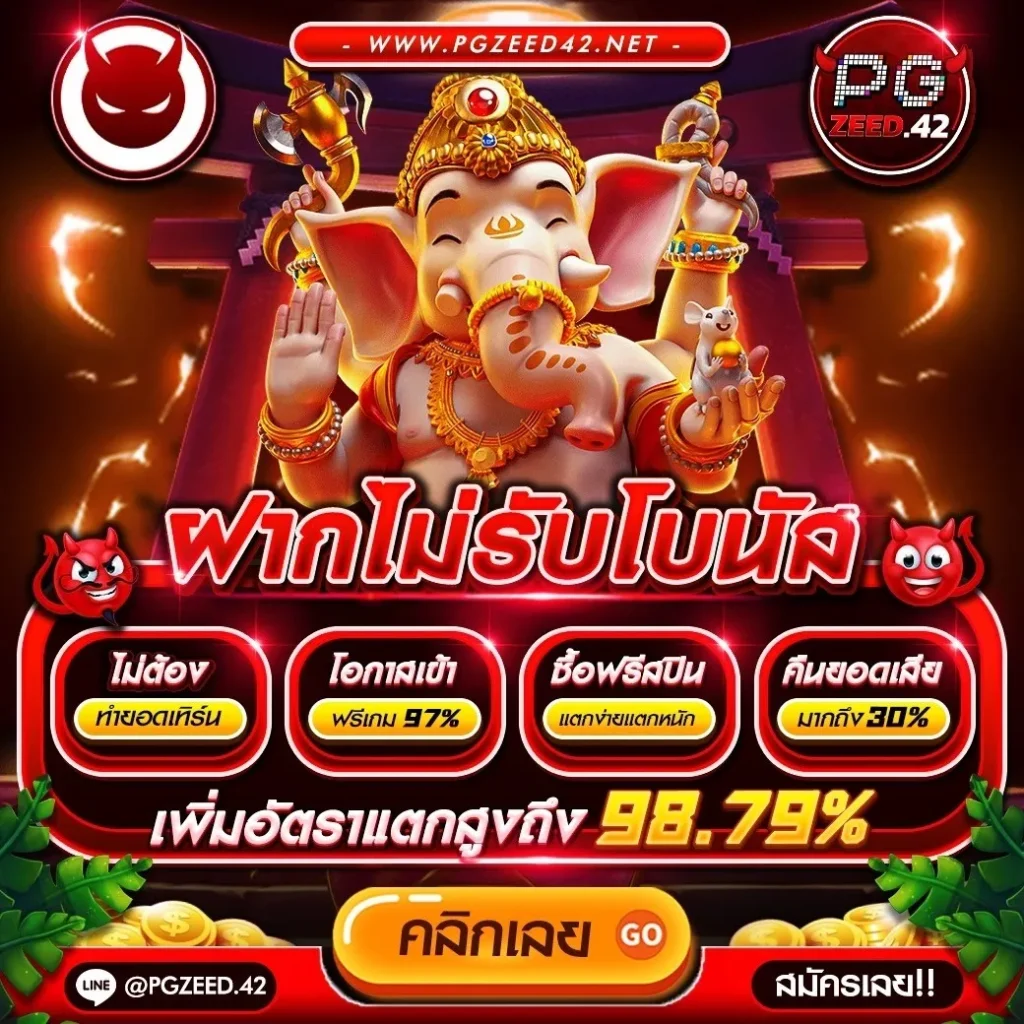
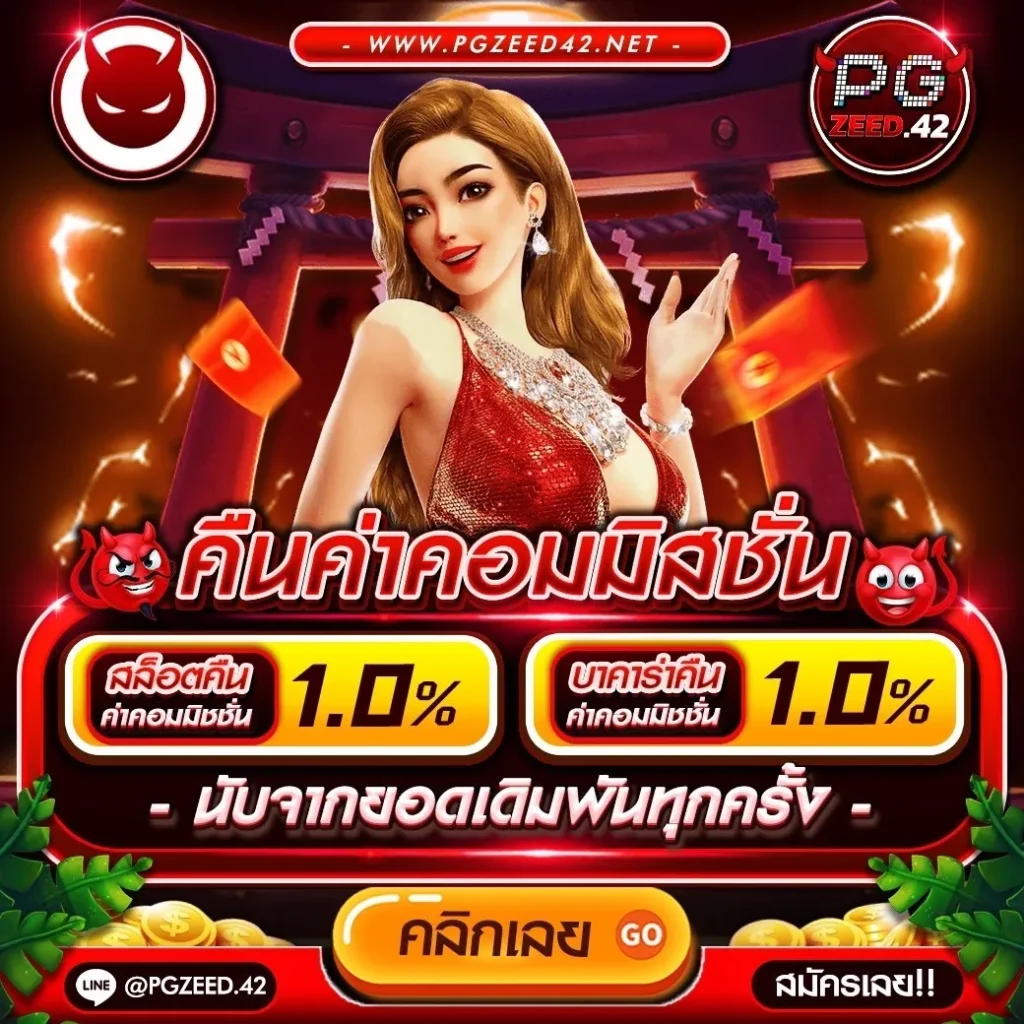
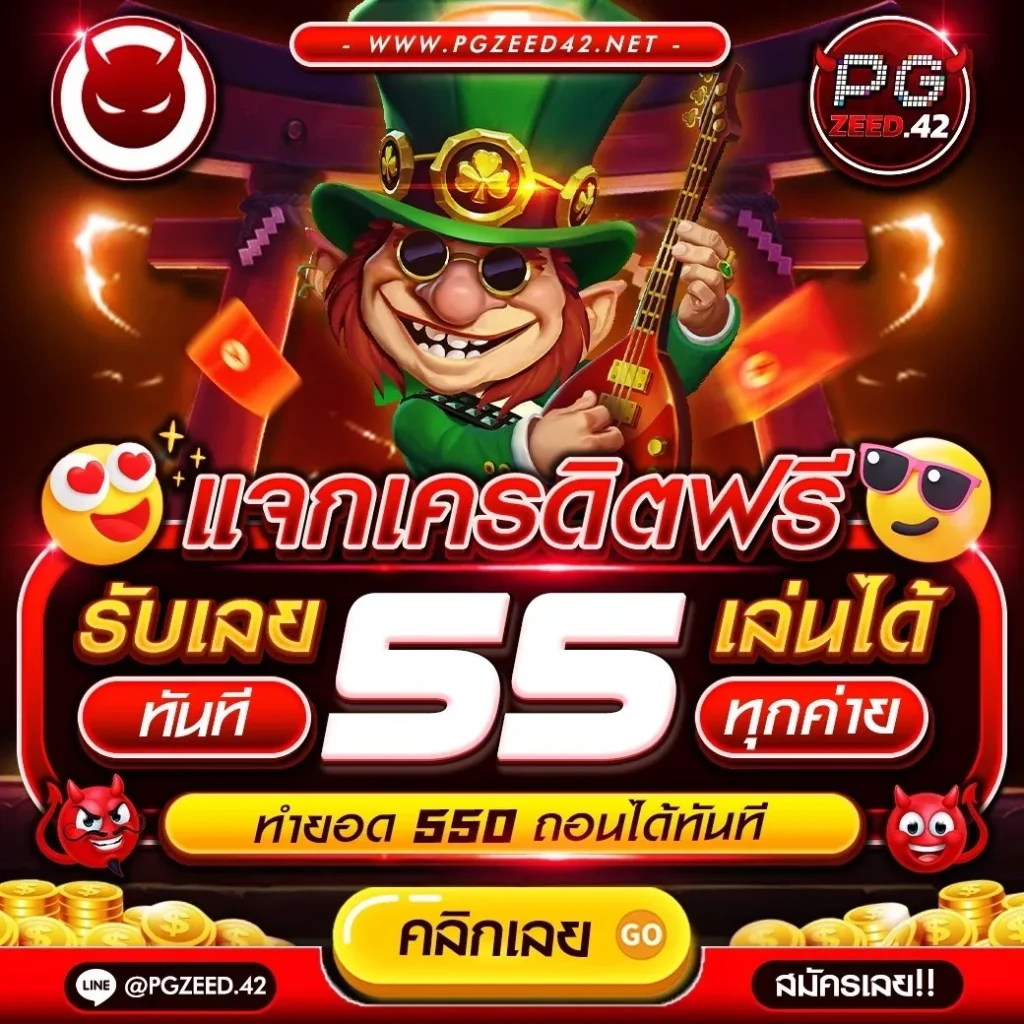
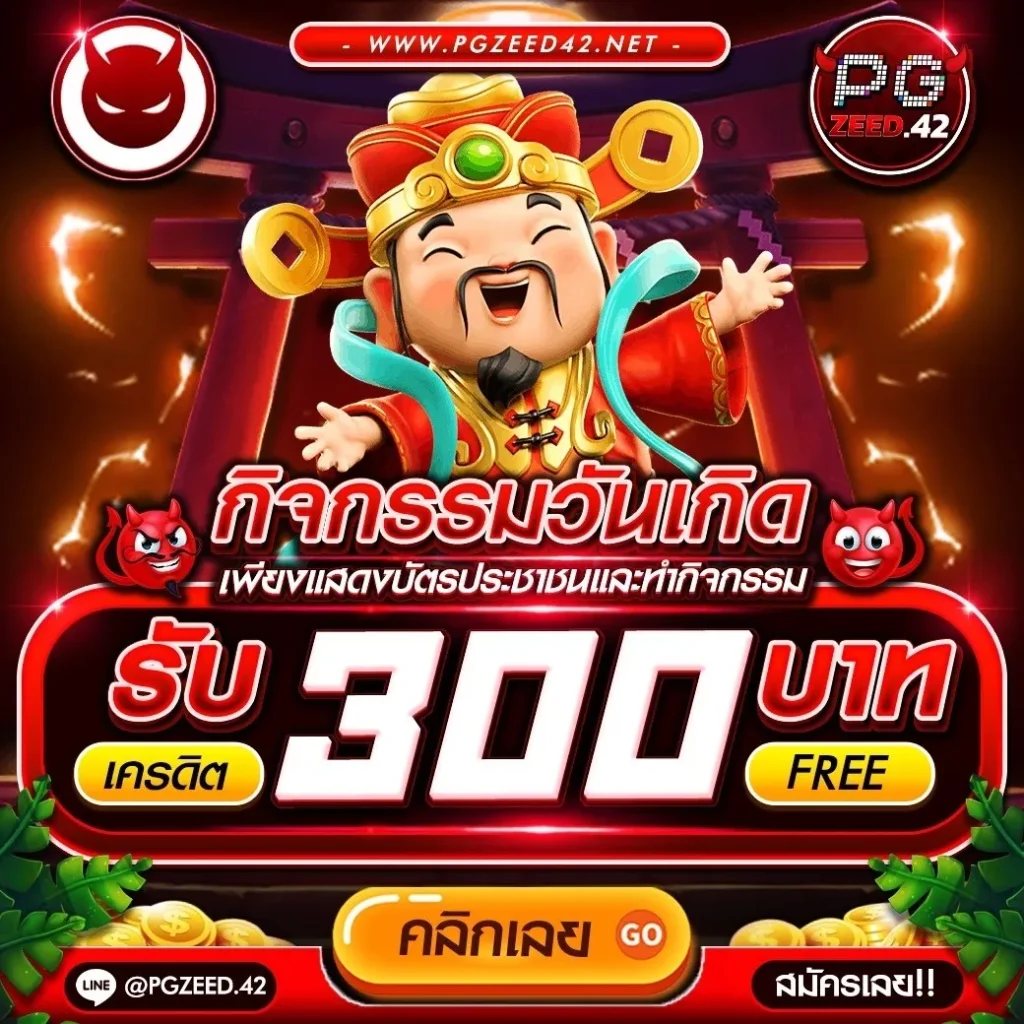
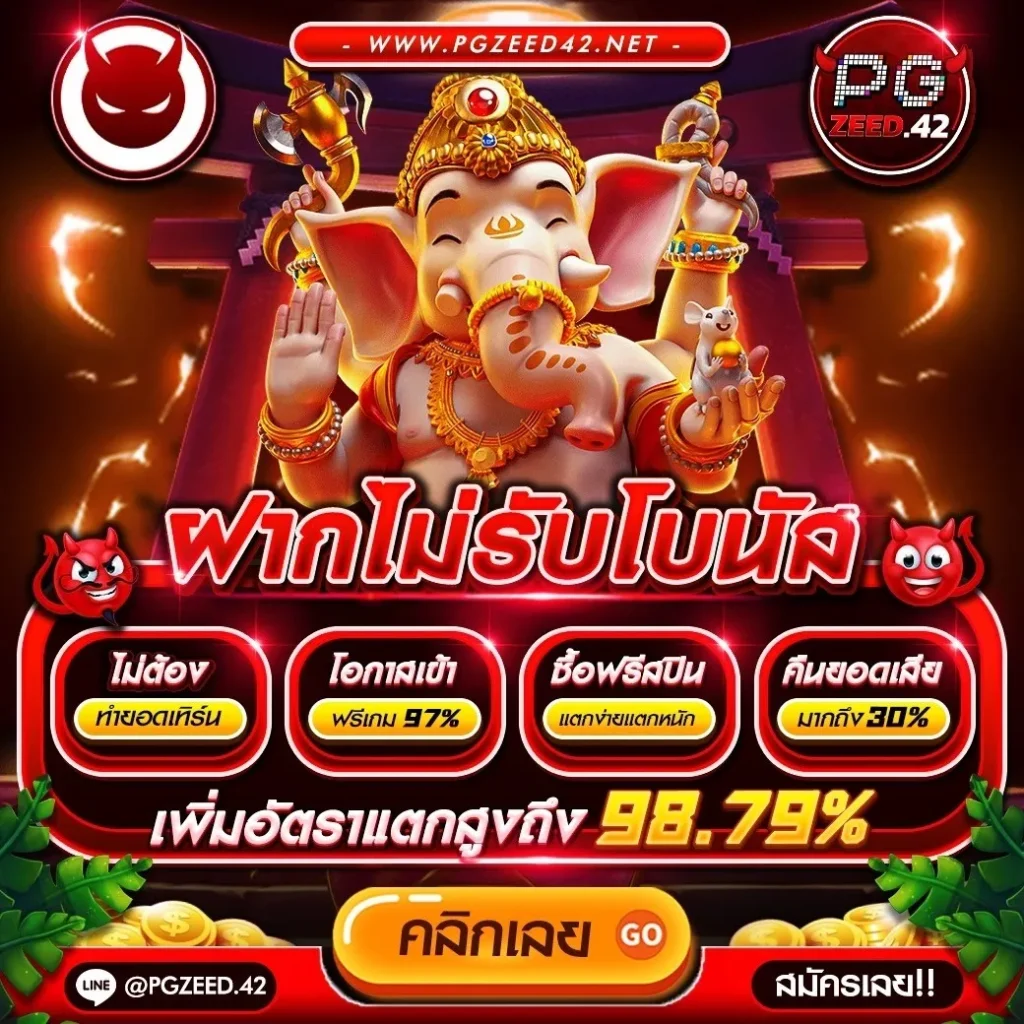
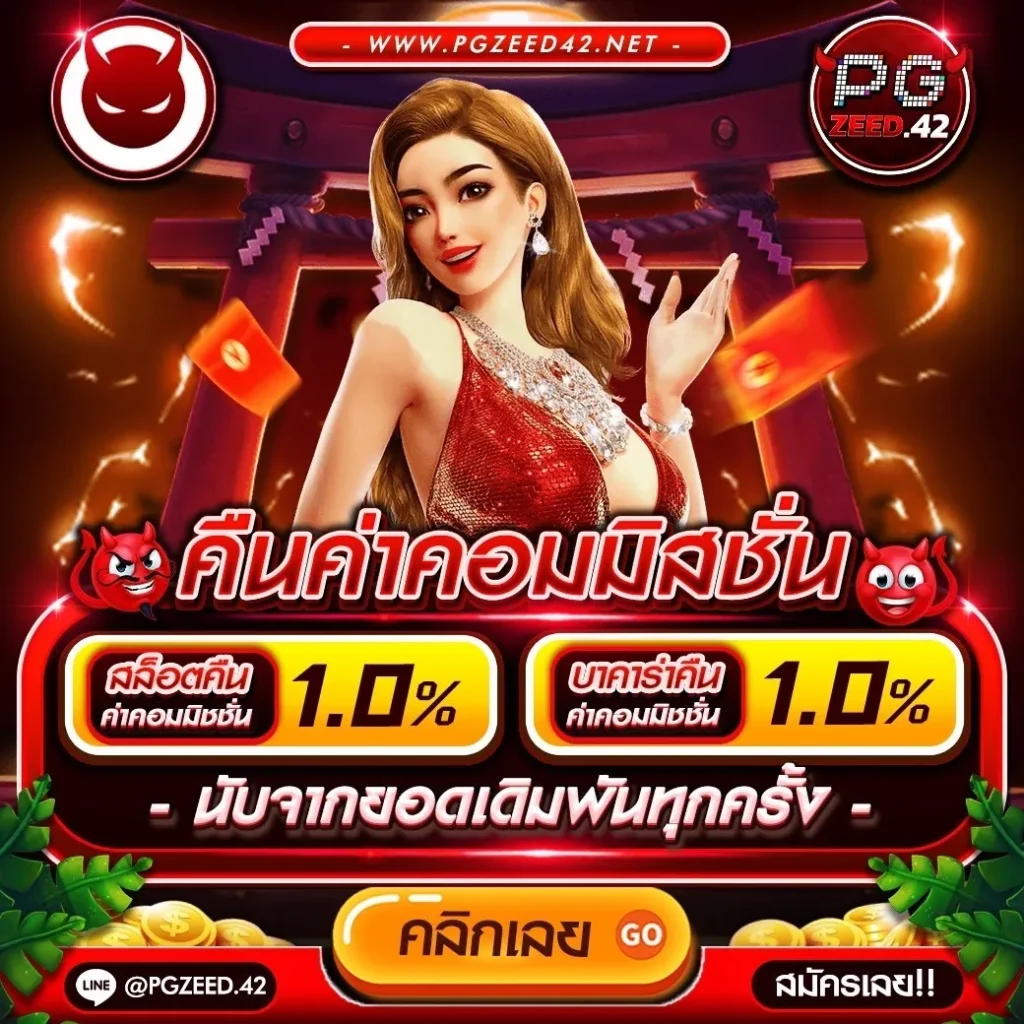
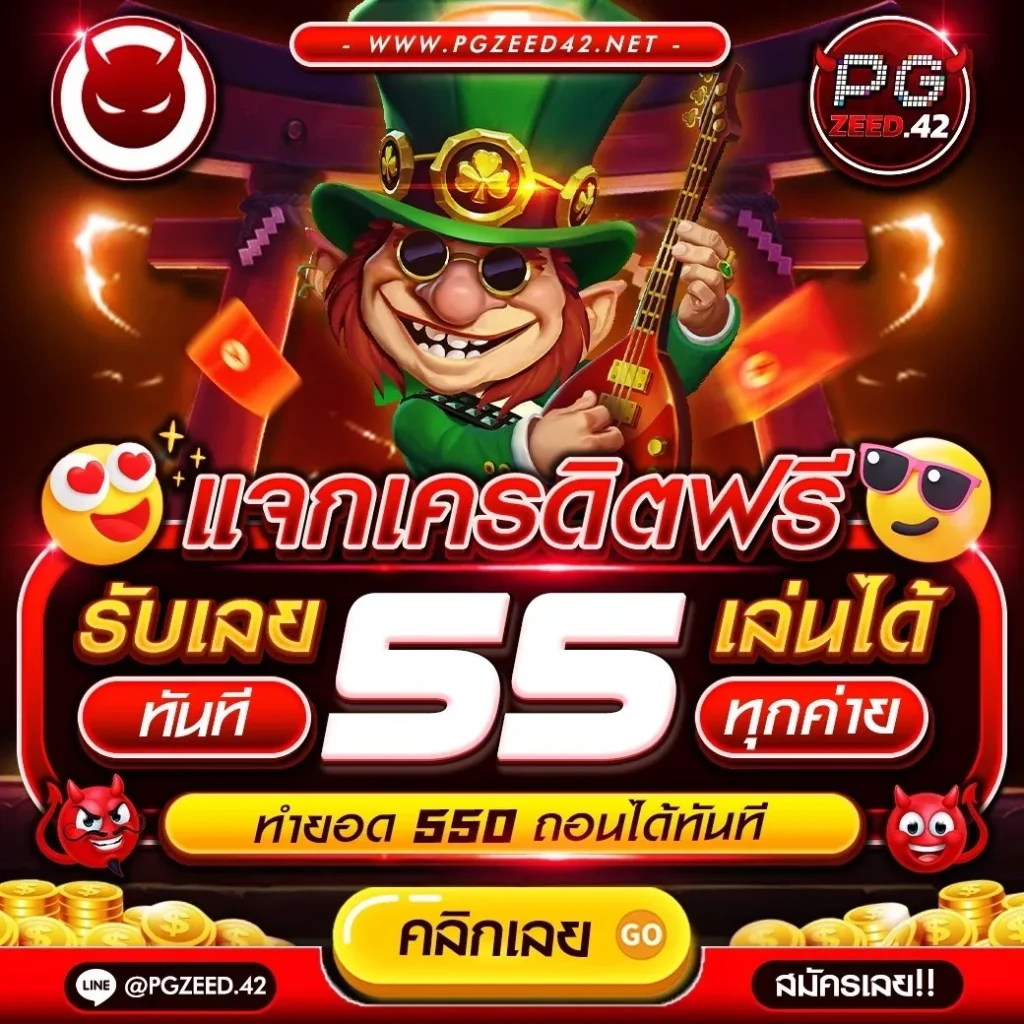
โปรโมชั่น pgzeed42 เว็บตรงออนไลน์

โปรโมชั่นพิเศษสำหรับสมาชิก pgzeed42 อาจรวมถึงข้อเสนอต่างๆ ออกแบบมาเพื่อปรับปรุงประสบการณ์การเล่นเกมสล็อตและเพิ่มมูลค่าให้กับผู้เล่น ไม่ว่าคุณจะเป็นสมาชิกเก่าหรือไม่ก็ตาม ทุกคนที่เล่นเป็นครั้งแรกสามารถพูดได้ว่าเว็บไซต์ของเราเสนอข้อเสนอฟรีเพื่อให้ทุกคนสามารถวางเดิมพันได้ฟรีโดยไม่ต้องทำการถอนขั้นต่ำ การบริการทุกระดับก็น่าประทับใจ PGZEED24 ผู้ดูแลระบบพร้อมให้บริการทุก 24 ชั่วโมง สามารถสอบถามโปรโมชั่นต่างๆ
- โบนัสต้อนรับสำหรับผู้เล่นใหม่ เพื่อดึงดูดผู้เล่นใหม่ มักจะมีโบนัสพิเศษ เช่น โบนัสการฝากครั้งแรกหรือการหมุนฟรีสำหรับเครื่องสล็อตบางเครื่อง
- โปรโมชั่นตามเทศกาลหรือโอกาสพิเศษต่างๆ จัดโปรโมชั่นตามเทศกาลต่างๆ เช่น ปีใหม่ วันหยุดสำคัญ หรือกิจกรรมพิเศษอื่นๆ
- โบนัสเงินฝาก โบนัสเพิ่มเติมเมื่อทำการฝากเงิน เช่น รับเปอร์เซ็นต์เพิ่มเติมจากจำนวนเงินที่ฝาก
- รางวัลแจ็คพอต เครื่องสล็อตบางเครื่องมีรางวัลแจ็คพอตขนาดใหญ่ เพิ่มโอกาสในการชนะรางวัลใหญ่
pgzeed42 เว็บตรงเปิดใหม่บริการ 24 ชั่วโมง

PG SLOT เป็นชื่อที่รู้จักกันดีในวงการสล็อตออนไลน์ ด้วยการนำเสนอเกมคุณภาพสูงที่ใช้เทคโนโลยีล่าสุด PGZeed ได้สร้างประสบการณ์การเล่นเกมที่ไม่เหมือนใครซึ่งดึงดูดผู้เล่นทุกระดับทักษะ หนึ่งในไฮไลท์ของ PGZeed คือการออกแบบและกราฟิกที่สวยงามและทันสมัย
เกม pgzeed42 แต่ละเกมมีธีมและเรื่องราวที่เป็นเอกลักษณ์ซึ่งทำให้ไม่เพียงแต่เล่นสนุกเท่านั้น แต่ยังสนุกกับการเล่นอีกด้วย แต่ยังรวมถึงการเสพติดความสวยงามของภาพและเสียงด้วยเกมเหล่านี้นำเสนอวิธีการชนะที่หลากหลายรวมถึงคุณสมบัติเพิ่มเติมที่น่าสนใจ
สิ่งที่ทำให้ PGZeed แตกต่างคือคุณสมบัติพิเศษและโบนัสต่างๆ ที่มีให้ในแต่ละเกม ไม่ว่าจะเป็นการหมุนฟรี รอบโบนัสพิเศษ หรือแจ็คพอตคุณภาพสูง PGZeed มอบทุกสิ่งเพื่อมอบประสบการณ์ที่ไม่เหมือนใครให้กับผู้เล่น PGZeed ไม่ได้เป็นเพียงชื่อในอุตสาหกรรมสล็อตออนไลน์ pgzeed42